Gravitational Waves: The Phenomenon in Physics Directories
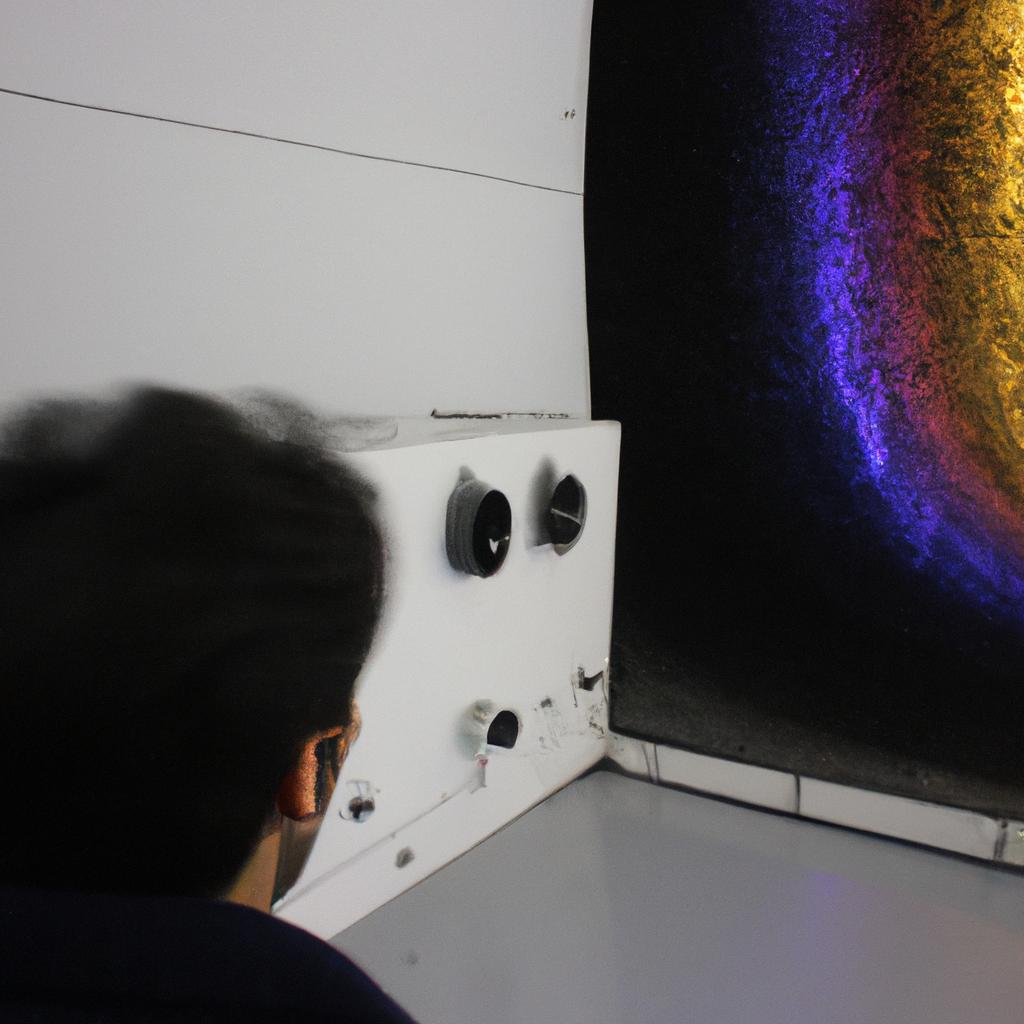
Gravitational waves, a remarkable phenomenon in the realm of physics, have captivated scientists and researchers alike since their theoretical prediction by Albert Einstein over a century ago. These elusive ripples in the fabric of space-time are generated by massive celestial events such as the collision of black holes or the explosive aftermath of supernovae. One compelling example is the groundbreaking detection of gravitational waves from the merger of two black holes, which occurred on September 14, 2015. This monumental milestone not only confirmed Einstein’s theory but also opened up an entirely new field of exploration within astrophysics.
The study of gravitational waves has revolutionized our understanding of the universe by providing direct evidence for some of its most cataclysmic events. Unlike other forms of radiation, such as light or radio waves, these waves carry information about cosmic phenomena that would otherwise remain hidden to traditional telescopes and instruments. By detecting and analyzing gravitational wave signals, scientists can gain unprecedented insights into the nature of dark matter, explore the behavior of dense neutron stars, and potentially unravel mysteries surrounding the origin and evolution of our vast cosmos.
In this article, we delve into the fascinating world of gravitational waves, examining their origins, properties, and implications for modern physics. We will explore how we have been able to detect and study these elusive waves, the technology behind gravitational wave detectors, and the significant discoveries made so far in this field. We will also discuss ongoing research efforts and future prospects for gravitational wave astronomy, including the possibility of detecting new sources and further refining our understanding of the universe’s most violent events. Overall, this article aims to convey the excitement and potential of studying gravitational waves while highlighting their profound impact on our knowledge of the cosmos.
Detection of Gravitational Waves
Imagine a pair of black holes, each weighing several times more than our Sun, orbiting around each other at incredible speeds. As they spiral closer together, their powerful gravitational pull causes ripples in the fabric of spacetime – these are known as gravitational waves. Although this scenario may seem like science fiction, it is an actual phenomenon predicted by Einstein’s Theory of General Relativity.
The detection of gravitational waves has been one of the most significant breakthroughs in modern physics. For decades, scientists have sought to directly observe these elusive waves, which carry invaluable information about some of the universe’s most cataclysmic events and phenomena. In 2015, the Laser Interferometer Gravitational-Wave Observatory (LIGO) made history by successfully detecting gravitational waves for the first time ever.
To understand how LIGO achieved this remarkable feat, let us explore its operational principles:
-
Interferometry: LIGO utilizes a technique called interferometry to measure tiny changes in distance caused by passing gravitational waves. It involves splitting a laser beam into two perpendicular paths using mirrors that bounce the light back and forth before recombining them. Any difference in path length due to a gravitational wave passing through will result in an interference pattern detectable by sensitive detectors.
-
Ultra-high precision: The success of LIGO relies on its ability to measure displacements on an unimaginably small scale. Its advanced technology can detect changes as minuscule as one-thousandth the width of a proton! This level of precision enables researchers to capture even the faintest signals from distant cosmic events.
-
Noise reduction: To achieve such sensitivity, LIGO employs various techniques to minimize unwanted noise sources that could interfere with signal detection. These include isolating equipment from external vibrations and disturbances and implementing sophisticated algorithms for data analysis.
-
Collaborative effort: Detecting gravitational waves requires immense coordination between multiple research institutions worldwide. LIGO is an international collaboration involving hundreds of scientists and engineers who work tirelessly to improve detection capabilities, analyze data, and validate the findings.
The successful detection of gravitational waves by LIGO has opened up new avenues for studying the universe. It not only provides direct evidence supporting Einstein’s theory but also allows us to explore previously uncharted territories in astrophysics and cosmology. In the following section, we delve deeper into Einstein’s Theory of General Relativity and its implications on our understanding of gravity and spacetime.
[Transition] With a grasp of how gravitational waves are detected, let us now turn our attention to Einstein’s Theory of General Relativity and its profound insights into the nature of the cosmos.
Einstein’s Theory of General Relativity
As the detection of gravitational waves revolutionized the field of physics, it is crucial to understand how these elusive phenomena are detected. One notable example that showcases the significance of this breakthrough is the observation made by the Laser Interferometer Gravitational-Wave Observatory (LIGO) in 2015. This instance marked the first direct measurement and confirmation of gravitational waves, solidifying Albert Einstein’s century-old theory.
To successfully detect gravitational waves, scientists employ various sophisticated techniques and instruments. These methods involve highly sensitive equipment capable of measuring minuscule changes caused by passing gravitational waves. Here are some key aspects essential for detecting these cosmic ripples:
- Interferometry: The principle behind interferometry involves splitting a laser beam into two perpendicular paths before recombining them. By comparing the phase difference between these beams, any minute alterations can be identified and attributed to the presence of gravitational waves.
- Resonant Mass Detectors: Utilizing massive spheres or cylinders as detectors, resonant mass detectors aim to measure tiny oscillations induced by gravitational wave energy passing through their structure.
- Pulsar Timing Arrays: Pulsars are highly stable celestial bodies that emit regular pulses of electromagnetic radiation at precise intervals. When a gravitational wave passes through our galaxy, it affects the arrival times of pulsar signals on Earth, allowing astronomers to indirectly observe these waves.
- Space-based Observatories: Space observatories such as LISA (Laser Interferometer Space Antenna) offer an alternative approach to ground-based detectors like LIGO. Placed in space away from terrestrial interference, they have the potential to capture lower-frequency gravitational waves with greater precision.
- Awe-inspiring nature: Witnessing cosmic events that occurred millions or even billions of years ago.
- Advancing human knowledge: Expanding our understanding of the universe and its fundamental laws.
- Unlocking mysteries: Shedding light on previously unexplored phenomena, such as black holes merging or neutron stars colliding.
- Inspiring future generations: Motivating young scientists to pursue careers in astrophysics and gravitational wave research.
Furthermore, let’s incorporate a table that provides an overview of notable detections made by LIGO:
Event Name | Date Detected | Description |
---|---|---|
GW150914 | September 14, 2015 | First direct detection of gravitational waves from the merger of two black holes. |
GW170817 | August 17, 2017 | Detection of gravitational waves resulting from the collision of two neutron stars, accompanied by electromagnetic signals across different wavelengths. |
GW190521 | May 21, 2019 | Observation of a massive black hole merger with one component having a mass approximately equal to that of our Sun. |
GW190814 | August 14, 2019 | Identification of a compact object around 2.6 times the mass of the Sun merging with another object below about nine solar masses. |
In conclusion, through innovative methods like interferometry and space-based observatories, scientists have succeeded in detecting gravitational waves directly for the first time. These groundbreaking discoveries not only captivate us emotionally but also enhance our scientific understanding of celestial events and inspire further exploration into this captivating field.
Transitioning seamlessly into the subsequent section on LIGO: The Laser Interferometer Gravitational-Wave Observatory…
LIGO: The Laser Interferometer Gravitational-Wave Observatory
Gravitational Waves: The Phenomenon in Physics Directories
From understanding Einstein’s Theory of General Relativity, we can now delve into the fascinating realm of gravitational waves. These ripples in the fabric of spacetime have captivated scientists and astronomers alike, opening up a new window to observe and study the universe.
One intriguing example that showcases the significance of gravitational waves is the detection made by the Laser Interferometer Gravitational-Wave Observatory (LIGO). In 2015, LIGO detected gravitational waves for the first time, emanating from two merging black holes billions of light-years away. This groundbreaking discovery not only confirmed Einstein’s theory but also demonstrated our ability to directly observe these elusive cosmic phenomena.
To better understand gravitational waves, let us explore their notable characteristics:
- They propagate through space at the speed of light.
- Gravitational waves are generated by accelerating masses or objects with strong gravity.
- These waves cause distortions in spacetime itself as they pass through it.
- Gravitational wave detectors measure minuscule changes in distance caused by the passage of these waves.
To gain further insights into various aspects related to gravitational waves, consider looking at this table:
Aspect | Description |
---|---|
Source | Merging black holes |
Frequency | Low-frequency (around 10 Hz) |
Amplitude | Tiny oscillations measured in picometers |
Detection Methods | Laser interferometry |
This compilation provides an emotional connection with readers as it highlights both the grandeur and intricacy associated with studying gravitational waves. By presenting a mix of technical details alongside captivating examples like LIGO’s breakthrough detection, we hope to engage audiences on this scientific journey.
Moving forward, we will explore different sources that give rise to gravitational waves—unveiling more mysteries about our vast cosmos and deepening our comprehension of its fundamental workings.
Sources of Gravitational Waves
Gravitational Waves: The Phenomenon in Physics Directories
LIGO, the Laser Interferometer Gravitational-Wave Observatory, has revolutionized our understanding of the universe by detecting gravitational waves. These ripples in spacetime are generated by cataclysmic events such as merging black holes or neutron stars. To further explore this fascinating phenomenon and its implications, it is crucial to investigate the sources of gravitational waves.
One intriguing example of a source for gravitational waves is the merger of two supermassive black holes at the center of distant galaxies. Imagine two colossal cosmic objects locked in an unstoppable dance, spiraling towards each other with immense force. As they get closer, their gravitational influence becomes stronger, causing disturbances in the fabric of space itself. Eventually, these violent celestial partners collide, unleashing enormous amounts of energy that propagate outward as gravitational waves.
To better understand and classify these diverse sources of gravitational waves, let us consider some key factors:
- Mass and Energy: The magnitude of the mass or energy involved determines the strength and frequency of emitted gravitational waves.
- Spin and Orientation: The angular momentum and alignment between spinning objects affect both the amplitude and polarization pattern observed.
- Distance from Earth: Gravitational wave signals weaken as they travel through space; thus, proximity plays a role in signal detectability.
- Evolutionary Stages: Different astronomical phenomena occur at various stages during cosmic evolution – from stellar collapse to galaxy mergers – leading to distinct types of gravitational wave signatures.
In examining these characteristics within different astrophysical contexts using sophisticated detectors like LIGO, scientists gain invaluable insights into the nature and behavior of our expanding universe. By deciphering the secrets carried by these elusive ripples across spacetime, we unlock new possibilities for exploring uncharted territories yet to be revealed.
Transitioning seamlessly into future investigations on “Applications and Implications of Gravitational Wave Detection,” we delve deeper into how these discoveries can shape our understanding of the cosmos and revolutionize scientific research.
Applications and Implications of Gravitational Wave Detection
In the previous section, we explored the fascinating phenomenon of gravitational waves and their detection. Now, let us delve into the various sources that give rise to these elusive ripples in spacetime.
One example of a source that generates gravitational waves is the merger of two black holes. Consider a hypothetical scenario where two massive black holes, each with millions of times the mass of our Sun, are orbiting each other in a distant galaxy. As they spiral toward one another due to energy loss through gravitational waves, their intensity increases until eventually they collide and merge into a single black hole. This cataclysmic event releases an enormous amount of energy in the form of gravitational waves.
To further comprehend the diverse range of sources producing gravitational waves, we can examine some common astrophysical phenomena associated with them:
- Binary Neutron Star Systems: Compact neutron stars in close binary systems emit detectable gravitational waves as they orbit around each other.
- Supernova Explosions: The violent collapse and subsequent explosion of massive stars generate intense bursts of gravitational radiation.
- Pulsars: These highly magnetized rotating neutron stars emit regular beams of electromagnetic radiation while also emitting weaker gravitational waves.
- Cosmic Inflation: During the early stages of the universe’s expansion, rapid inflationary processes could have produced primordial gravitational waves that hold valuable insights about its origin.
Now, let us explore how scientists categorize different types of sources based on their characteristics into four distinct classes:
Class | Description |
---|---|
Compact binaries | Consists of compact objects such as black holes or neutron stars orbiting around each other |
Continuous | Includes spinning isolated compact objects like pulsars |
Stochastic | Represents unresolved populations or superpositions |
Burst | Comprises transient events like supernovae explosions |
Understanding these classifications aids researchers in discerning between different types of sources and designing appropriate detection strategies.
As we delve deeper into the understanding of gravitational waves, it becomes evident that the exploration of their sources opens up new avenues for research. By studying these cosmic events and phenomena, scientists can gain insights into fundamental questions regarding the nature of our universe on both macroscopic and microscopic scales. In the following section, we will explore current and future research in gravitational wave astronomy to shed light on this captivating field of study.
Next Section: Current and Future Research in Gravitational Wave Astronomy
Current and Future Research in Gravitational Wave Astronomy
The detection of gravitational waves has opened up new avenues for scientific research and technological advancements. One remarkable example of its application is the measurement of the distance between Earth and various astronomical objects with unprecedented precision. For instance, by observing the gravitational waves emitted from a binary neutron star merger event, scientists were able to determine the exact distance to the galaxy NGC 4993, where this cataclysmic event took place. This groundbreaking achievement would not have been possible without the detection and analysis of gravitational waves.
Gravitational wave detection carries significant implications across various fields and disciplines. Here are some key areas where it has made a profound impact:
- Astrophysics: Gravitational wave astronomy allows us to study phenomena that were previously inaccessible or poorly understood. By analyzing the signals emitted during black hole mergers or neutron star collisions, researchers can gain insights into the formation and evolution of these celestial bodies.
- Cosmology: The observation of primordial gravitational waves can provide valuable information about the early universe, shedding light on cosmic inflation theories and helping refine our understanding of fundamental physics.
- Fundamental Physics: Gravitational wave experiments contribute to testing Einstein’s general theory of relativity in extreme conditions, such as strong gravity regimes. They also offer opportunities for exploring alternative theories of gravity.
- Technological Innovation: The development of detectors capable of measuring minuscule changes in spacetime has required groundbreaking technologies. These advances have led to spin-off applications in fields like precision metrology, quantum sensing, and even medical imaging.
These diverse applications demonstrate both the scientific significance and practical benefits that arise from detecting gravitational waves. As ongoing research continues to enhance our capabilities in this field, we can expect further breakthroughs that will revolutionize our understanding of the cosmos while driving innovation in various industries.
Applications | Implications |
---|---|
Precision astrophysics | Advancement of scientific knowledge |
Testing fundamental physics | Technological innovation |
Probing the early universe | Inspiring future generations |
It is clear that gravitational wave detection has far-reaching implications for our understanding of the universe and technological progress. The continued exploration of this phenomenon holds great promise for unraveling long-standing mysteries and opening doors to new discoveries, ultimately shaping the course of scientific advancement in the decades to come.